Approaches complementary to animal experimentation in agronomy and veterinary clinics: solutions and limits
Header
The use of animal models in biological research raises many questions for society in general, but also for those involved in this research. In previous centuries, it took a long time for animals to be recognised as sentient beings. Changes in social attitudes from antiquity to the present day have made this recognition possible. The regulatory framework for animal experimentation has evolved considerably over the last twenty years, in tandem with an ethical debate that has led to the creation of ethics committees since 1990. There is still room for improvement, but the implementation of the 2010 European Directive should contribute further to improving the condition of animals in experimental research. The aim of this review is to outline the various stages that led to the notion of 'animal consciousness', defined as the subjective experience that animals have of their environment, their own bodies and/or their own knowledge, and the need to develop new tools to reduce animal experimentation.
Introduction
Man's perception of animals has been strongly influenced by philosophical theories, the most important of which defended the idea that it is consciousness, in other words the soul, that differentiates man from animals. These theories have continued to evolve over the centuries and, since Antiquity, have played an important role in the experimental methods available to mankind to explore living organisms and, in particular, the brain, which was very early on considered to be the seat of consciousness.
The development of complementary or alternative methods to animal experimentation, which also enable to reduce the number of animals used in animal experimentation in research, and particularly in agronomic research, responds to ethical, societal and scientific concerns. The development of new technologies (cell culture, organ-on-chip, organoids) provides innovative experimental solutions for the study of animal physiology and pathologies, with their strengths and limitations. The aim of this summary is firstly to outline the history of the awakening of animal consciousness, through the philosophical and biological currents that for centuries opposed each other on the status of the animal, and then through the domestication of animals, which was a key element in the use of animals by man. We will then look at the various stages of animal protection legislation, that led to laws aimed at reducing the use of animals for scientific purposes. Finally, we will look at the development of alternatives and complementary experiments to animal experimentation, from the discovery of the cell to the creation of mini-animal organs.
1. History of animal awareness and ethics - The human-animal relationship
Animal consciousness has long been a subject of research for scientists and philosophers. As far back as antiquity, philosophers such as Aristotle, the Epicureans and the Stoics believed that non-human animals were not subject to justice (there were no penalties for mistreatment) because they lacked reason, while other philosophers such as Porphyry (Porphyry, 1979) and Plutarque (Plutarque, 2002) asserted that animals were sentient and should therefore be respected. A few centuries later, René Descartes (Descartes, 1973) and Emmanuel Kant (Kant, 1986) stated that animals lacked consciousness, while Michel de Montaigne (De Montaigne, 1962), Jean-Jacques Rousseau (Guichet, 2002) and (Bentham, 1823) recognised that animals were sentient and even social beings. However, it was only at the end of the twentieth century that animal ethics (a branch of philosophy devoted to the moral responsibilities of human beings towards animals of other species, viewed individually) was established as a philosophical discipline in its own right - more precisely in 1975, the year of publication of the seminal work Animal Liberation, by the Australian philosopher Peter Singer (Singer, 2012). Peter Singer pioneered animal ethics by combining a philosophical approach with scientific demonstrations to highlight animal suffering and the abusive use of animals in animal experimentation. He thus introduced a pathocentric perspective, according to which individuals have a non-instrumental value because they are sentient. It rejects the notions of speciesism and utilitarianism, which portray animals as having a status inferior to humans. Two collective expert reports have been published on pain and animal consciousness, bringing together philosophers and biologists. An initial collective scientific assessment conducted by INRAE (Institut national de recherche pour l'agriculture, l'alimentation et l'environnement) on behalf of the French Ministries of Agriculture and Research on "Animal pain in livestock farming" (Le Neindre et al., 2009) was followed in 2017 when INRAE (via its Department of Collective Scientific Expertise, Foresight and Studies) took up these issues by conducting a collective scientific assessment on animal consciousness, at the request of the European Food Safety Authority (EFSA). This work led to a clarification of the concepts and analysis methods used to understand pain in livestock. This work has also made it possible to characterise and identify painful situations. Painful interventions may be carried out on animals to meet the constraints of certain farming systems, but also to meet the requirements of the organoleptic quality of a product or worker safety. Slaughtering conditions, for example, have been specifically studied. It is well known that stress at slaughter has a major impact on meat quality (particularly in pigs), and this work has helped to identify solutions to reduce or even eliminate pain and stress at slaughter. The results were presented to representatives of the member countries of the European network on animal welfare and the EFSA on 11 May 2017 in Parma (Italy) (Le Neindre et al., 2017).
Another process in the evolution of consideration for the animal condition is animal domestication, which responds to a utilitarian ethic. Animal domestication dates back to the Palaeolithic period with the domestication of the dog, the first animal to have been domesticated (-40,000 years ago) (Druzhkova et al., 2013). This was followed by other species brought by settlers in the Neolithic period: the cow, pig, sheep, goat and horse (9,600 years ago). This stage of domestication led to the development of agriculture and new eating habits (and a change in human lifestyle through sedentarisation) (Gautier, 1991). Then, around 5000 BC, it was the turn of poultry, in Asia, with the chicken. Finally, in the Middle Ages, rabbits and donkeys were domesticated, followed by other birds around 1500 AD (Chansigaud, 2020). All these practices are sometimes to the detriment of the animal, which loses genetic diversity and brain capacity and acquires traits that are sometimes to its disadvantage (Chanvallon, 2009). The process of domesticating species has led to physiological changes in animals (reduced aggressiveness, reduced brain volume, changes in seasonality) and certain practices have become dependent on humans, such as reproduction and horse riding (Chapouthier, 2009).
Figure 1. The human-animal relationship through the ages, including domestication - because this process is the basis for the 'use' of animals by humans.

The dog was the first domesticated animal and the grey wolf is thought to be the ancestor of the domestic dog. 99% of their DNA is common. The horse was domesticated not once, but at least twice. The first evidence of the domestication of the horse dates back around 5,500 years, the second around 2,200 BC. This was followed by the domestication of herbivores, notably cattle, and then chickens around 6,000 BC. The cat was domesticated around 50 BC, followed by the rabbit and the donkey in the Middle Ages. The turkey was domesticated later, around 1500.
2. Reconciling animal protection and the need to acquire knowledge: A body of regulations in France and Europe
Consideration of the interests of animals by legislators is a fairly recent development in human history. It began in 1804 with the creation of the Civil Code by Napoleon, in which animals were considered as "movable property" (art. 528) and over which the owner exercised a right of ownership. In France, the concern to protect animals did not receive the backing of the law until 1850, and then only in a very summary way, when on 2 July the National Legislative Assembly passed the Grammont Law: "Those who publicly and abusively mistreat domestic animals will be punished by a fine of between five and fifteen francs, and may be imprisoned for between one and five days". On 14 March 1861, the Court of Cassation defined domestic animals as "animate beings that live, are raised, are fed and reproduce under man's roof and by his care". Another important date was 7 September 1959, when the decree repealing and replacing the Grammont law was issued. The ill-treatment of animals was also penalised in the private sphere. The Act of 19 November 1963 created the offence of cruelty, followed by the Act of 10 July 1976, which set out the fundamental principles of animal protection: animals are sentient beings and must be kept in conditions compatible with their biological needs; it is forbidden to mistreat animals; it is forbidden to use animals improperly (Code rural et de la pêche maritime, article L. 214-1 to L.214.3).
The Council of Europe took an interest in animal protection (1960-1970), setting up conventions to protect farm, experimental and domestic animals. In 1987, the European Convention for the Protection of Pet Animals made a general reference to the welfare of domestic animals. In 1997, the Treaty of Amsterdam defined animals as "sentient beings for whom the implementation of Community policy in the fields of agriculture, transport, the internal market and research must take account of their welfare requirements". In 1999, following a new animal protection law, the French Civil Code was amended so that animals, while remaining property, were no longer treated as things.
Subsequently, article 521-1 of the Criminal Code "protects animals as sentient beings by imposing severe penalties for serious cruelty to animals under human care". In 2005, a report commissioned by the Minister of Justice recommended that "the Civil Code should recognise that animals are sentient living beings, and that the legal regime to be adopted for them should be deduced from this basic qualification". Then in September 2010, in the European directive on animal experimentation, the notion of sentience appeared once again: "Animals should therefore always be treated as sentient creatures". A bill on animal protection was tabled on 13 November 2012, which included Article 515-14 stipulating that "Animals are sentient living beings. They must be kept in conditions consistent with the biological requirements of their species and respect for their welfare". On 16 April 2014, the first passage of the bill on the modernization and simplification of the law and procedures in the fields of justice and home affairs took place in the hemicycle of the National Assembly. The deputies voted in favor of the Glavany amendment, which included article 515-14 as follows: "Animals are sentient living beings. Subject to the laws that protect them, animals are subject to the regime of tangible property". On 30 October 2014 the MPs adopted the text of the law, including the provision recognizing animals as sentient living beings. Animals are recognized as sentient living beings. Their legal status (movable or immovable property) remains unchanged and the rules governing their ownership continue to apply.
This symbolic measure is viewed positively by animal associations and animal lovers, who see it as a first step towards changing the place accorded to animals in our society. https://www.fondation-droit-animal.org/informations-juridiques/textes-relatifs-a-lanimal/
Then recently, the Act of 30 November 2021 was passed to combat animal mistreatment and strengthen the bond between animals and humans.
Figure 2. Key stages in animal protection regulation
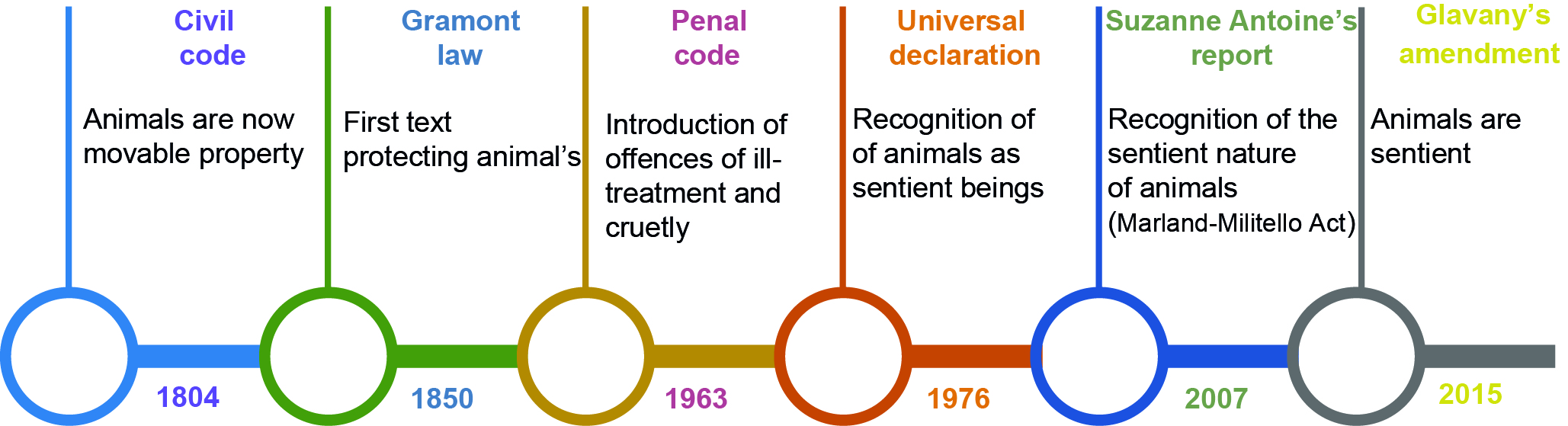
Timeline of key stages in the recommendations and laws enacted in France from 1804 to the Glavany amendment of 2015.
2.1. How can livestock farming be repositioned in this context?
The domestication of animals has turned farmed animals into "utility" animals, and today's livestock farming is the result of this age-old process. At present, the intentional objectives of domestication or the improvement of domestic breeds essentially concern production (rarely the work produced by the animals). These are adaptations to farming conditions, prolificacy, growth rate, and often the quality of meat or other products such as milk or wool. The first records showing the formalisation of animal selection by lineage date back to the 16th century BC (Sanchez et al., 2000). Modern selection requires objective evaluation of animals and rigorous organisation of breeding programmes to improve the performance of lines in line with specific objectives. The most advanced form of domestication corresponds to intensive breeding, where the breeder provides everything necessary for the development of the animals, to maximise their production or enable them to be reared on small areas. In livestock farming, the intensification that accompanies modernisation tends to reduce the direct interaction between farmer and animal (Fiorelli et al., 2012).
However, for several decades now, the human-animal relationship and the welfare of farm animals have become a subject of great importance in agronomy (Boivin et al., 2012).
2.2. Animal experimentation in question or Questioning animal experimentation?
The use of animals to acquire and increase knowledge of biological, physiological and medical processes dates back to antiquity. Roman law prohibited the dissection of human cadavers, and Galen (around 130-200 AD) can be credited with the systematic practice of animal experimentation, mainly on pigs and monkeys. Progress in scientific knowledge has led to a better understanding of how the bodies of animals and humans 'work'. This progress is based on experimental biological research itself, the principles of which were described in the nineteenth century by Claude Bernard (Bernard, 1952), and whose foundations are post-Cartesian: living bodies, as material systems, can be analysed and understood through experimentation. These advances in scientific knowledge have demonstrated the extraordinary similarity between the way animal and human bodies function. The development of the use of animals in biomedical research was marked in the 19th century by Claude Bernard, a fervent defender of the experimental method. In "Introduction to the Study of Experimental Medicine", published in 1865, he wrote: "We can only learn about the laws and properties of living matter by dislocating living organisms in order to gain access to their inner environment". This experimental method heralded a new era in medicine. And it enabled Claude Bernard to make major discoveries. Most of his experiments involved vivisection. "Even his wife and daughters, fierce animal rights activists, ended up turning their backs on him" (Corvol, 2012).
Despite the long history of animal experimentation, no regulations, either legislative or moral, were put in place until the 20th century. Since the reform of the penal code in 1994, these acts of vivisection of animals have been punishable and come under the category of "other crimes and offences". What's more, the penalties for animal abuse have just been strengthened in the 2018 EGALIM law. In fact, there is a significant difference to be taken into account between mistreatment (unjustified and therefore unacceptable) and use for scientific purposes (utilitarian approach, inducing pain/suffering greater than that of a needle) assessed by an ethics committee. It has become imperative to guarantee animals still being used for legitimate reasons a level of protection and welfare as high as the objectives of the experiment allow. In addition, it is important to know the assessment of the ratio relationship between the damage caused to the animals and the expected benefits of the experiment.
The moral concern shared by many people not to cause animals to suffer led, on the one hand, to the creation of associations (the Société Protectrice des Animaux created in France in 1845, and the Animaliste party founded in 2016, the first French political party dedicated to the defence of animals) and, on the other, to the introduction of a law to protect animals into the penal code in 1850 (the Grammont law).
In animal experimentation, the issue of pain management has led to the establishment of the 3S rule. Members of the INRAE group of experts suggest using the 3S rule: suppress, substitute, relieve. The first question is whether the potentially painful procedure is really necessary, and if so, whether it should be carried out using the least painful method (Le Neindre et al., 2009). Finally, if the latter does nevertheless cause pain, it should be relieved using early, multimodal and appropriate analgesia: i) removing the source of pain when it does not provide any benefit to the animals or the farmers; ii) replacing a technique that causes pain with another method; iii) relieving unavoidable pain using pharmacological treatments.
3. The 3Rs and 3Ss: new stages!
3.1. The "3Rs" rule, Development of alternative methods to respond to societal concerns around an ethical approach to animal experimentation
In 1959, Russell and Burch described the "3Rs" principle - Replace, Reduce, Refine - for research using animals (Tannenbaum and Bennett, 2015). This principle recommends substituting the use of higher-consciousness animals (live vertebrates) with more primitive life forms or with in vitro experiments and computer simulations. The principle of reduction recommends that research and procedures should be carried out with as few animals as possible, while the principle of refinement suggests that the techniques used should reduce their pain and distress at all stages of the study (Miziara et al., 2012; Petroianu, 1996).
3.2. “Alternative methods" to animal experimentation
The term "alternative methods" was introduced in 1978 by David Smyth (Smyth, 1978). It covers methods that satisfy one or more of the "3Rs" principles (Replace - Reduce - Refine).
In 2012, OPAL (an association founded in 1968 on the use of animals in animal experimentation) organized a symposium focusing on the 4th R: "Responsibility of all those involved in animal experimentation". Those involved in animal research have a duty to apply ethical principles in order to carry out high-quality research while protecting the animals as much as possible. The majority of society's expectations regarding the use of alternative methods in experimentation appear to be well-founded and consistent with the progress of research, analysis of the issues at stake and regulatory developments.
3.3 Official information on alternative methods
The European Centre for the Validation of Alternative Methods (ECVAM): https://eurl-ecvam.jrc.ec.europa.eu/ is a European reference laboratory. In addition, the national platform for the development of alternative methods in animal experimentation (FRANCOPA) is dedicated to the development and dissemination of alternative methods in animal experimentation, created in 2007: http://www.francopa.fr/web/francopa?page=home&out=txt&languageIhm=fre.
Finally, the FC3R (France Centre 3R) was created in 2021 to support the application of the rules stemming from the 3R principle at the request of the Ministry of Higher Education, Research and Innovation, in the form of a Scientific Interest Group (GIS). It has a wide range of missions and resources. The aim of this structure is to be recognised in France and Europe as a reference and contact point for all issues relating to the 3Rs, in both public and private research. The founding members of the GIS are Inserm, CNRS, INRAE, Inria, CEA, Institut Pasteur de Paris, CPU and Udice. https://presse.inserm.fr/creation-dun-groupement-dinteret-scientifique-reference-francaise-pour-toutes-les-questions-relatives-aux-3r-2/44037/.
The objectives of the FC3R are : to achieve a significant reduction in the number of animals used in experiments, particularly through longitudinal multimodal approaches, to ensure better replacement by invertebrate models or complementary approaches, particularly in vitro; i) - to ensure refinement based on innovative practices; ii) to ensure training in rigorous and responsible practice, in line with the 3Rs principle, for all students and/or new entrants required to use animals for scientific purposes; iii) to position the FC3R as a key player in France and Europe in the development of alternative methods to the use of animals and in other issues relating to the 3Rs.
A major book on alternatives to animal experimentation, which explains the advances made in this area, was published very recently and is proving to be a useful tool for improving visibility on the subject (Marano et al., 2020).
The German non-governmental organization "Doctors Against Animal Expriments" has created a database of alternative methods to animal experimentation for research purposes. The database is called NAT Database (NAT for Non animal technologies) and is available in English and German. The search criteria are as follows: field of research concerned, method, year and country of publication.
3.4. The political turn in animal ethics
In accordance with the principles of European Directive 2010/63/EU, France has set up an institutional framework for research using animals for scientific purposes. Ethics committees were first set up in Canada in 1968, before being introduced in France in 1980 (in industry and the armed forces) and 2001 in public research. The Ministry of Research issues authorizations for projects using animals, after a favorable ethical assessment of the project by the Animal Experimentation Ethics Committees (C2EA), which carry out an ethical assessment based on the determination of a cost/benefit balance between the potential benefits of the project and the expected harm to the animals. In France, two authorities predominate: Agriculture and Research. Agriculture has a regalian mission of controlling structures and compliance with regulations (approval, compliance of projects, skills of people). This task is delegated to the DGAL and the DDPP. Research is responsible for the ethical assessment and authorization of projects. The Commission Nationale de Protection et d'Utilisation d'Animaux à des Fins Scientifiques (CNPAFIS) is placed under the aegis of the 2 ministries (decree 2013/118).
This guarantees the regulatory compliance of the infrastructures and operations of the establishments, in particular those of the Structures in charge of Animal Welfare (SBEA), which ensure the operational monitoring of authorized projects and the dissemination and promotion of the 3Rs rule (Replace, Reduce and Refine) within the establishments. https://www.sbea-c2ea.fr/lanimal-dans-la-recherche/
Figure 3. System implemented in France in accordance with the principles of European Directive 2010/63/EU.
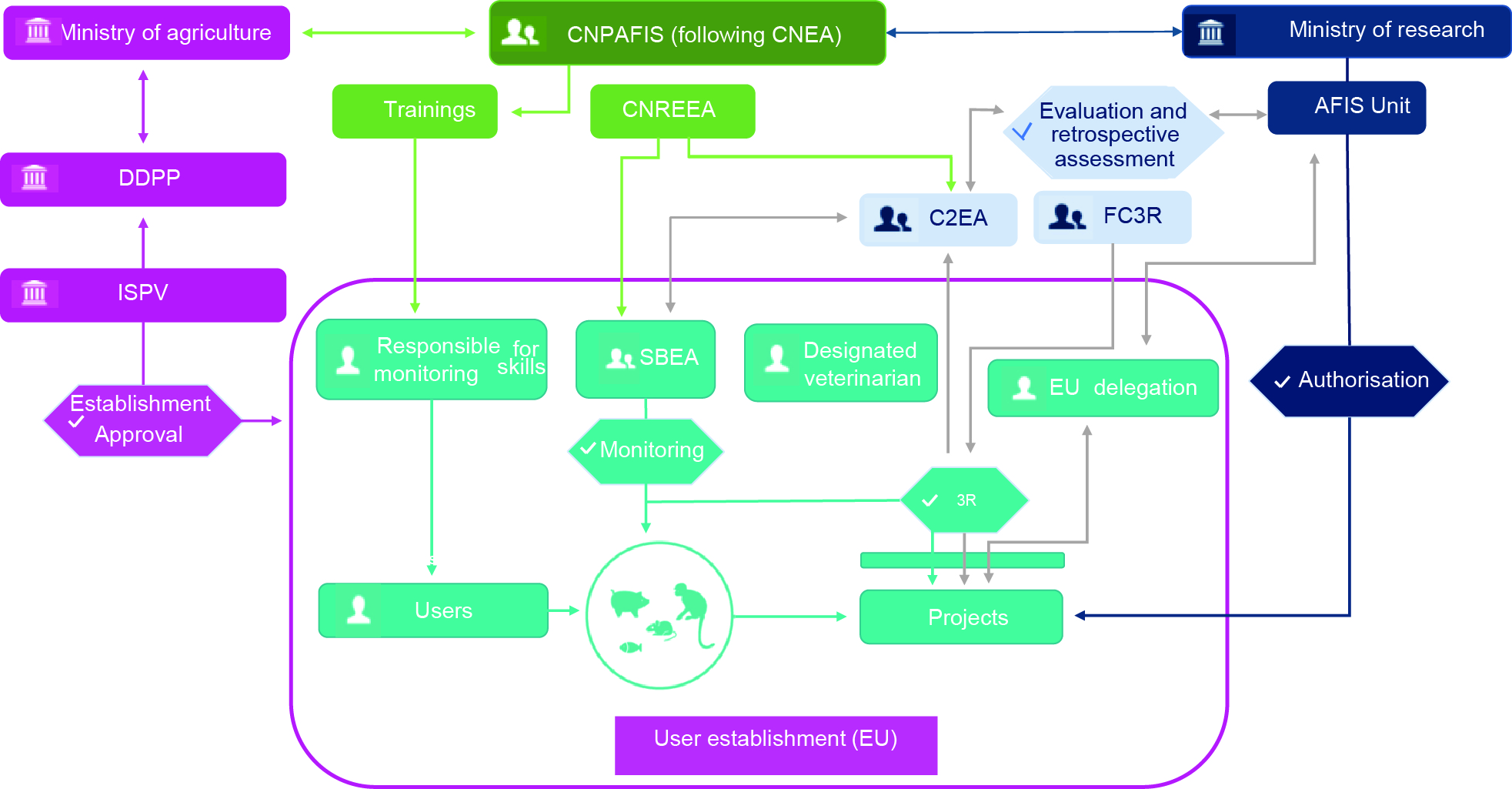
Institutional framework for research using animals for scientific purposes set up by France in accordance with the principles of European Directive 2010/63/EU.
4. What are the practical alternatives to animal experimentation?
The dialogue that has been established between these committees and researchers has led to the development of hitherto ignored principles such as the 3Rs (Replace, Reduce, Refine). This has led to improved experimental conditions, a gradual reduction in the number of animals through rational use and the replacement of animals by in vitro and in silico techniques, particularly at different stages of research.
4.1. In vitro approaches
Box 1. In vitro approaches.
In vitro approaches. Refers to chemical, physical or immunological reactions, or all experiments and research carried out in the laboratory, outside a living organism (tissue cultures, hormone synthesis, etc. are carried out in vitro).
The development of the biological sciences would not have been possible without one of the greatest inventions of all: microscopes. In the 16th and 17th centuries, two countries, the Netherlands and Italy, played a crucial role in the construction and use of microscopes and telescopes. In the Netherlands, around 1590, Hans Janssen and his son invented a microscope made of two convex lenses. In 1665, the English physicist Hooke published the first major work on the construction of the microscope, its components and microscopic observations. In his micrographs he illustrated the microscopic structures of numerous biological samples (insects, plants, sponges, bryozoans, fossils, etc.) observed under the microscope and described the microscopic units. “Cells" or "pores", as he called the small compartments in a slice of cork (thickened walls of dead cells), were chosen to designate these microscopic units. Although Robert Hooke used the term 'cell' differently from later biologists, today's term 'cell' comes directly from Hooke's Micrographia (Hooke, 1965). At the end of the 19th century, Wilhelm Roux (1885) demonstrated that it was possible to maintain living cells (from the neural plate of chicken embryos) outside the body in a saline buffer for a few days (Sander, 1991). The American embryologist Ross Granville Harrison (1870-1959) developed the first in vitro cell culture techniques at the beginning of the 20th century (Souza et al., 2016). Harrison's experiments made cell life "visible" by developing techniques for visualizing his cultures between slide and coverslip. In his research article "Observations on the Living Developing Nerve Fiber", he described a method for maintaining nerve cells and developing fibers ex vivo (Harrison, 1907). Unfortunately, Harrison's observations were limited in time by bacterial contamination. For this reason, Harrison introduced aseptic techniques for working with cell cultures. Glassware was flambéed, surgical equipment (needles, scissors and forceps) was boiled and tissues/filter papers were autoclaved. The aseptic technique resulted in sterile preparations that could be maintained in vitro for more than five weeks. As a result of these changes in sterile tissue preparation, Harrison was able to demonstrate various stages of cell development continuously over time (Nicholas, 1961). Through the development of his technique, Harrison highlighted enormous possibilities for the application of cell and tissue culture not only as a tool in bacteriology, embryology, physiology or histology studies, but also for the production of monoclonal antibodies, vaccines and drugs (Rodriguez-Hernandez et al., 2014). Subsequently, an experiment by Alexis Carrel (winner of the Nobel Prize in Physiology and Medicine in 1912) that had a major impact on his time was the chicken heart that he made beat in vitro, in a nutrient liquid, for several decades (an age that no chicken reaches) (Soupault, 1952). This opened the way to two areas of research: i) the conservation of living organs for possible transplantation purposes; and ii) the exact limit to the lifespan of different organs.
4.2. Cell lines
Cell lines are immortalized cells, usually by transfection, that multiply by mitosis and represent a homogenous population. Their major advantage is that they can be maintained in culture for a very long period of time.
In 1925, the ATCC (American Type Culture Collection), a world center for biological resources, was founded when a committee of scientists recognized the need for a centralized collection of micro-organisms that scientists around the world could use to conduct research to advance the science of microbiology. The collections later expanded to include cell lines and the ATCC became a standard-setting organization as well as the leading developer and supplier of authenticated cell lines and micro-organisms. In 1927, the first ATCC catalogue was published. The second edition two years later included 650 new cultures. https://www.atcc.org/.
The first cell line, the 'L' cell line, was established by Earle in 1948. This cell line was derived from mouse subcutaneous tissue and had a morphology quite different from the original tissue (Marquis, 2012).
In the 1950s and 60s, other diploid cell lines were developed such as HeLa, MRC-5 and WI-38 from human tissues (Rodriguez-Hernandez et al., 2014). The cell line called HeLa (derived from the patient's name - Henrietta Lacks), was cultured for one year and in 1952 Dr Gey and colleagues published the first results on this line (Gey et al., 1952). HeLa cells were shown to grow in a variety of media - chicken plasma medium, bovine embryo extract and human placental cord serum. The HeLa cell line established by Gey gave Jonas Salk and John Enders gave or provided the opportunity to develop poliovirus cultures in a non-nervous tissue system. Polio virus was also successfully propagated in HeLa cell cultures by Dr Gey (Lucey et al., 2009). HeLa cells have become the most popular and valuable resource for cancer studies (Meechan and Wilson, 2006).
4.3. However, the main limitations and disadvantages of using cell lines today are:
- Genetic aberrations in cell lines linked to an increase in the number of passages,
- Genotypic and phenotypic drift in lines, particularly those deposited in cell banks for many years,
- The different methods of culturing tumor and cancer cells (2D and 3D),
- Cross-contamination of cell cultures with the HeLa cell line (it has been reported that a large number of cancer cell lines are cross-contaminated),
- Culture conditions can modify morphology, gene expression and several cellular pathways,
- Mycoplasma infections can alter the properties of the culture, (Edmondson et al., 2014).
4.4. Stem cells
One of the most remarkable advances came in 1957 when the first human bone marrow transplant was attempted by Dr Edward Donnall Thomas, a medical researcher working in Seattle at the time who went on to win the Nobel Prize in 1990 for his work (Storb, 2012). Thanks to his research in immunology, Dr (Robert A.) Good was able to carry out the first allogeneic bone marrow transplant. Bone marrow transplantation is a form of haematopoietic stem cell transplantation in which haematopoietic stem cells are injected into a patient to treat various blood diseases, including certain autoimmune and hereditary diseases, and cancer. Dr Robert A. Good performed the first bone marrow transplant on a child suffering from an immune deficiency in 1968. The boy received bone marrow from his sister and continued to live into adulthood (O'Reilly, 2003).
In 2006, Shinya Yamanaka of Kyoto University in Japan obtained embryonic-type cells from adult cells, avoiding any ethical dilemma arising from the need to destroy an embryo (Takahashi and Yamanaka, 2006). Capable of reprogramming specialized adult cells into pluripotent cells by inserting 4 key genes (Oct3/4, Sox2, Klf4 and c-Myc), he was able to form what are now known as "induced pluripotent stem cells" (iPS). iPSCs are capable of self-renewal and differentiation into several cell types (Doi et al., 2009).
In 2012, the Nobel Prize in Physiology and Medicine was awarded to John B. Guordon (for the discoveries that proved the reversible nature of cell specialization) and Shinya Yamanaka (for the reprogramming of mature murine cells into immature cells) (Trounson and DeWitt, 2013). Both of these discoveries are of great importance in many areas of medicine, such as oncology and regenerative medicine. It has been reported that embryonic stem cells have been successfully used in cartilage repair, peripheral nerve repair or cardiac regenerative therapy. In addition, mesenchymal stem cells have been used in certain types of therapy, for example autologous transplants or therapies for haematopoietic diseases (Sykova and Forostyak, 2013).
Figure 4. The key stages in the development of cell cultures, from the discovery of the cell to the development of mini-organ.

The discovery of the microscope in 1665 revealed the presence of cells, and their study over the centuries has led to the development of new research tools that are still being perfected today.
4.5. New cell culture systems: current status, advantages and limitations
a. Organoids
Organoids are not simple cell cultures but are 3D structures derived from stem cells or progenitor cells that recreate important aspects of the 3D anatomy of the organ in miniature and may possess one or more tissue functions of the organ (Rossi et al., 2018). Organoids are defined by an in vitro three-dimensional cellular self-organization consisting of several cell types performing functions normally present in the organ in vivo.
Organoids can be generated from adult stem cells (ASC) (Sato et al., 2009), embryonic stem cells (ESC) (Eiraku et al., 2008) or iPSC cells (Takahashi and Yamanaka, 2006). For farm animals, organoids could be used for in vitro phenotyping, by testing the in vitro characteristics of organoids that can be an indicator of traits of interest (Archibald et al., 2020). Other applications include the study of pathologies and the mechanisms by which tissues function in response to certain factors.... The models currently being developed using species of agronomic interest are mainly those relating to embryonic development and the foetus-mother relationship, the physiology of tissues such as muscle tissue, adipose tissue, the mammary gland and the liver, tissue-microbiota interaction, especially in the intestine, but potentially in the skin, lung and any tissue in contact with a microbiota, and host-pathogen interaction, both bacterial and viral, in various tissues such as the intestine, skin, lung and brain.
In contrast to these models, that use animals of agronomic interest, organoids were first developed in rodents and humans, and the application of these new in vitro models to farm animals opens up opportunities to reduce animal experimentation and new prospects for predictive approaches for many species (rabbits, poultry, monogastric animals, ruminants, etc.). A special issue of Veterinary Research: https://www.biomedcentral.com/collections/organoids-animals was published in 2021, showing the strong development of these techniques in agronomy (Archer et al., 2021; Baquerre et al., 2021; Beaumont et al., 2021; Bourdon et al., 2021; Dessauge et al., 2021; Kar et al., 2021; Orr et al., 2021; Pain et al., 2021; Pain, 2021; Souci and Denesvre, 2021; Vermeire et al., 2021). For muscle, for example, Kim et al (2020) have developed an innovative 3D bioprinting system based on decellularised extracellular matrix cells that can be used to obtain the functions and mechanical properties of skeletal muscle tissue in vitro (Kim et al., 2020).
b. 3D bioprinting
Box 2. 3D bio-printing.
3D bioprinting refers to a type of 'additive' manufacturing, a layer-by-layer manufacturing technique that originated from a need for rapid 'custom' manufacturing and has since evolved into a rapid and customizable manufacturing method in many fields. 3D bioprinting technology allows flexibility in material choice and design paradigm - in the context of tissue engineering, the ability to incorporate biomaterials and cells intrinsically enables 3D bioprinting. As 3D bioprinting becomes more ubiquitous, more research into bioprinting techniques has emerged, enabling the fabrication of a wide range of biocompatible constructs, cell-encapsulated tissues and organ models (Xiang et al., 2022).
The development of the ECM (extracellular matrix) makes it possible to obtain cell-cell and ECM-cell interactions in cultures. Using 2D cell cultures, researchers have not been able to mimic the in vivo state. Conventional monolayer cultures have various limitations, for example the loss of tissue-specific architecture. The development of new techniques has made it possible to improve the microenvironment of cell cultures, for example, three-dimensional (3D) cell culture models. This technique has enabled scientists to produce non-adherent and adherent cell cultures. For non-adherent cells, cell aggregation can be achieved using Ultra Low Attachment (ULA) dishes and/or agarose and polyhydroxyethyl methacrylate (pMEMA) coated dishes. 3D cultures of adherent cells can be obtained using porous materials for prefabricated scaffolds that promote cell adhesion. The 3D culture format offers a unique opportunity to analyse and understand cell growth, migration and organisation (Mandrycky et al., 2016).
c. The 3D culture technique (organoids and 3D bioprinting) is based on the idea of mimicking and offers a number of advantages
- This model is more representative of in vitro conditions and has biochemical and morphological characteristics specific to the in vivo state,
- 3D culture ensures the cell-cell and cell-ECM interactions (mechanical and biochemical signals) that are essential for various processes such as differentiation and proliferation,
- The interactions between tissue-specific cells and the bloodstream are also important. The development of technologies to vascularize 3D cultures is essential in many studies and is expanding in relation to this point. In vitro vascularization strategies can be classified into modelling and self-organization methods (Nashimoto et al., 2017).
- This type of culture ensures more precise tissue-specific architecture,
- There are different types of 3D cell culture systems, for example, 3D spheroids grown on matrix, 3D spheroids grown in matrix (scaffold-based 3D culture), 3D spheroids grown in suspension, 3D cultures without scaffold. Unlike organoids, spheroids are simple clusters of cells, derived for example from hepatocytes, nervous tissue, skeletal muscle cells or mammary glands. They do not require scaffolding to form 3D cultures; the cells assemble spontaneously in clusters with each other. They cannot self-assemble or regenerate, so are not as advanced as organoids.
d. Limits and drawbacks
- In some 3D cultures, cell detachment is difficult,
- Some existing systems fail to mimic the biomechanical characteristics of tissues in vivo because they maintain a static condition,
- For scaffold-based growing systems, reproducibility between different batches is not satisfactory,
- In synthetic scaffolds based on PolyEthyleneGlycol (PEG), the PEG is compatible with the cells but the cells that are included are not able to bind to the matrix without modification,
- Recovery of cells encapsulated in a matrix or scaffold (e.g. for nucleic acid or protein isolation), screening and bioprocessing (thermal, filtration) in 3D culture systems such as imaging tools are difficult. For example, the autofluorescence of collagen scaffolds is troublesome for fine immunolabelling (Rimann and Graf-Hausner, 2012; Wu et al., 2019).
3D bioprinting technology is one of the most exciting innovations, but the idea of 3D printing is not new. The first description of 3D printing was made by Charles W. Hull, he called his method "sterolitography" (Hull, 2018). The formation of 3D scaffolds for biological materials was the first step in the development of this technology. The next step was to evaluate the technique that prints living cells layer by layer in 3D scaffolds (Murphy and Atala, 2014).
The success of the bioprinting process depends on the selection of cells for printing tissues or organs. Organ or tissue printing requires several cell types, for example, primary functional cells, embryonic and induced pluripotent stem cells. The cells chosen must be robust to survive the printing process, so in many studies cell lines are used. For example, fibroblasts or transformed cell lines are sufficiently robust to withstand shear stress and pressure (Mironov et al., 2002).
e. Ethical limits
Numerous ethical issues surrounding research on organoids and gastruloids have already been identified. The ethical problem arises with cerebral organoids (cerebroids) and the notion of "consciousness" of the structures formed. The creation of chimeras by xenotransplantation to ensure the vascularisation and innervation of organoids, by transplanting human cerebroids into the brains of adult animals, is not covered by regulations (Lavazza and Massimini, 2018). These manipulations pose a real ethical problem because experimentally it is possible to modify the cerebral activity of an animal transplanted with a human cerebral structure (Yeager, 2018). For example, a transplant of human glial cells can modify the cognitive functions of a mouse (McGinley et al., 2018). Many of these issues are not specifically addressed by existing ethics control structures, but these controls could be easily extended to ensure that organoid and related research progresses appropriately and ethically (Munsie et al., 2017). Members of the International Society for Stem Cell Research (ISSCR) have drafted new guidelines for stem cell research to reflect advances in stem cell science and other areas of research that need to be protected and framed on ethical, social and political issues that have arisen since the last update in 2016. These guidelines include scientific and ethical guidance for the transfer of human pluripotent stem cells and their derivatives into animal models (Lovell-Badge et al., 2021).
INSERM's ethics committee has also examined the ethical issues involved in organoid research, which currently has no legal framework (https://www.hal.inserm.fr/inserm-02544395/document).
Another ethical issue concerns the use of foetal calf serum in cell culture. It is highly controversial because of the method used to produce these sera, and many substitutes (human plasma (Vlaski-Lafarge et al., 2019), synthetic sera, etc.) are being developed to avoid using animal serum.
f. Modelling: in silico methods and the contributions of "omics" (genomics, transcriptomics, proteomics, metabolomics, etc.)
In silico methods are based on the development of computer simulation tools.
The study of omics, or high-throughput studies, is expanding rapidly in a number of areas, from the oldest, such as genomics and transcriptomics, to the most recent, such as microbiomics and exposomics, providing a wealth of data that can be used in silico. Omics, combined with powerful statistical data processing, make it possible to obtain a large amount of information from a single sample (which makes it possible to reduce the number of samples, in line with the 3Rs).
The contributions of omics, combined with bioinformatics, are the result of the need to process genomic information and the strong appropriation of computer technologies by researchers. To facilitate access to and processing of biological sequences, it has been necessary to record them in banks, now online on the Internet. These sequence banks are filtered by teams of bioinformaticians to create data warehouses dedicated to specialized research on an organism, a type of molecule, etc. (Gallezot, 2002). This gives access to a large amount of data that can be processed for different research themes without duplicating experiments.
In agronomy, for example, a study used in silico approaches to understand the growth of Staphylococcus aureus on products made from pork, and thus demonstrated that the bacterium grows faster in ham than in sausage, this being linked to the effect of temperature (Tango et al., 2015). In silico methods combined with omics data enable mechanistic studies as well as gene and protein linkage studies to study a function.
g. The scientific community
A major advantage at present is that scientific societies are getting organised. For example, the Groupement de Recherche sur les Organoïdes (GDR, 2102) (https://gdr-organoides.cnrs.fr/) was set up in 2021 by the CNRS under the leadership of Dr Vincent Flacher, and brings together scientists from a number of French research institutes. Its aim is to support this field of research, enabling members of the scientific community and industries to exchange ideas and structure the field in France. At INRAE, the Organoids group led by Bertrand Pain enables members of the same scientific community to discuss the development of tools for veterinary medicine and agronomy.
Bringing together experts on the extraordinary potential of these fast-growing technologies for fundamental and applied research in the fields of life sciences, human and animal health and well-being is vital to its progress.
Conclusion
According to Directive 2010/63/EU3: "While it is desirable to replace the use of live animals [...] it remains necessary to protect human and animal health and the environment". This shows the extent of the work that remains to be done to further reduce the number of animals used in animal experiments. However, this summary shows the efforts made by the scientific community to develop new alternatives and meet both regulatory and societal expectations. It is important to specify that fundamental research is based on the most relevant models, whereas in translational research (e.g. agronomy), researchers use the species concerned by their application. Work on livestock is therefore essential to answer questions relating to the species concerned, and there are many examples showing that it is still complicated to transpose results obtained on a model species to animals of an agronomic species. Thus, in agronomy and veterinary medicine, physiological and genetic studies using 3D in vitro models are being developed for the study of animals and offer many advantages. Other methods are also being developed, such as in silico studies, modelling and the integration of large datasets (big data) to reduce the number of animals used in animal experiments.
Acknowledgements
This article was first translated with www.DeepL.com/Translator and the authors thank Myriam Grundy for English language editing.
References
- Archer F., Bobet-Erny A., Gomes M., 2021. State of the art on lung organoids in mammals. Vet. Res., 52, 77. doi:10.1186/s13567-021-00946-6
- Archibald A.L., Daetwyler H.D., Groenen M.A.M., Harrison P.W., Houston R.D., Kühn C., Lien S., Macqueen D.J., Reecy J.M., Robledo D., Watson M., Tuggle C.K., Giuffra E., 2020. From FAANG to fork: application of highly annotated genomes to improve farmed animal production. Genome Biol., 21, 285. doi:10.1186/s13059-020-02197-8
- Baquerre C., Montillet G., Pain B., 2021. Liver organoids in domestic animals: an expected promise for metabolic studies. Vet. Res., 52, 47. doi:10.1186/s13567-021-00916-y
- Beaumont M., Blanc F., Cherbuy C., Egidy G., Lisabetta E., Lacroix-Lamandé S., Wiedemann A., 2021. Intestinal organoids in farm animals. Vet. Res., 52, 33. doi:10.1186/s13567-021-00909-x
- Bentham J., 1823. An Introduction to the Principles of Morals and Legislation, Oxford, Clarendon Press. https://global.oup.com/academic/product/the-collected-works-of-jeremy-bentham-9780198205166?cc=fr&lang=en&
- Bernard C., 1952. Introduction à l'étude de la médecine expérimentale [Texte imprimé] / Claude Bernard ; présentation de Constant Bourquin. Éditions Flammarion, Paris, France. 302 p.
- Boivin X., Bensoussan S., L'Hotellier N., Bignon L., Brives H., Brule A., Godet J., Grannec M.L., Hausberger M., Kling-Eveillard B.F., Tallet C., Courboulay V., 2012. Hommes et animaux d’élevage au travail : vers une approche pluridisciplinaire des pratiques relationnelles. In : Numéro spécial, Travail en élevage. Hostiou N., Dedieu B., Baumont R. (Eds). INRAE Prod. Anim., 25, 159-168. doi:10.20870/productions-animales.2012.25.2.3205
- Bourdon G., Cadoret V., Charpigny G., Couturier-Tarrade C., Dalbies-Tran R., Flores M.J., Froment L., Raliou M., Reynaud K., Saint-Dizier M., Jouneau A., 2021. Progress and challenges in developing organoids in farm animal species for the study of reproduction. Vet. Res., 52, 42. doi:10.1186/s13567-020-00891-w
- Chansigaud V., 2020. Histoire de la domestication animale. Éditions : Delachaux & Niestlé-, 400p. https://www.livres-medicaux.com/vie-animale/20864-histoire-de-la-domestication-animale.html
- Chanvallon S., 2009. Anthropologie des relations de l’Homme à la Nature : la Nature vécue entre peur destructrice et communion intime. 1180p. https://books.google.fr/books/about/Anthropologie_des_relations_de_l_Homme.html?id=uC28ZwEACAAJ&redir_esc=y
- Chapouthier G., 2009. Le statut philosophique de l'animal : ni homme, ni objet. Le Carnet PSY, 139, 23-25. https://www.cairn.info/revue-le-carnet-psy-2009-8-page-23.htm
- Corvol P., 2012. https://destinationsante.com/claude-bernard-le-pere-de-la-methode-experimentale.html
- Descartes R., 1973. Discours de la Méthode. (Eds) Livre de Poche. https://1000idcg.com/discours-de-la-methode/
- Dessauge F., Schleder C., Perruchot M.H., Rouger K., 2021. 3D in vitro models of skeletal muscle: myopshere, myobundle and bioprinted muscle construct. Res 52, 72. doi:10.1186/s13567-021-00942-w
- Doi A., Park I.H., Wen B., Murakami P., Aryee M.J., Irizarry R., Herb B., Ladd‐Acosta C., Rho J., Loewer S., Miller J., Schlaeger T., Daley G.Q., Feinberg A.P., 2009. Differential methylation of tissue‐ and cancer‐specific CpG island shores distinguishes human induced pluripotent stem cells, embryonic stem cells and fibroblast. Nature Genet., 41, 1350-1353. doi:10.1038/ng.471
- Druzhkova A.S., Thalmann O., Trifonov V.A., Leonard J.A., Vorobieva N.V., Ovodov A.D., Graphodatsky A.S., Wayne R.K., 2013. Ancient DNA Analysis Affirms the Canid from Altai as a Primitive Dog. PLoS ONE 8, e57754. doi:10.1371/journal.pone.0057754
- Edmondson R., Jenkins Broglie J., Adcock A.F., Yang J., 2014. Three‐dimensional cell culture systems and their applications in drug discovery and cell‐based biosensors. ASSAY and Drug Dev.Technol.,12, 207-218. doi:10.1089/adt.2014.573
- Eiraku M., Watanabe K., Matsuo-Takasaki M., Kawada M., Yonemura S., Matsumura M., Wataya T., Nishiyama A., Muguruma K., Sasai Y., 2008. Self-organized formation of polarized cortical tissues from ESCs and its active manipulation by extrinsic signals. Cell Stem Cell 3, 519-532. doi:10.1016/j.stem.2008.09.002
- Fiorelli C., Louret S., Porcher F., 2012. Les rationalités du travail avec les animaux d'élevage : produire, vivre ensemble et se construire. In : Numéro spécial, Travail en élevage. Hostiou N., Dedieu B., Baumont R., (Eds). INRAE Prod. Anim., 25, 181-192. doi:10.20870/productions-animales.2012.25.2.3207
- Gallezot G., 2002. La recherche in silico. In : Chartron G. (Ed). Les chercheurs et la documentation numérique : nouveaux services et usages, Édition du cercle de la Librairie, Collections. https://archivesic.ccsd.cnrs.fr/sic_00177318v1
- Gautier A., 1991. Domestication animale et animaux domestiques prétendument oubliés, Université de Liège, 1991, (ISSN 077724913 (http:/ /worldcat.org/issn/077724913&lang=fr)). cité ici (http://promethee.philo.ulg.ac.be/zoologica/lbodson/bibl/Resumes03.html).
- Gey G.C., Coffman W.D., Kubicek M.T., 1952. Tissue culture studies of the proliferative capacity of cervical carcinoma and normal epithelium. Cancer Res., 12, 264-265.
- Guichet J., 2002. L'homme et la nature chez Rousseau : L'homme de la nature, un homme absolument isolé ou détenteur déjà d'une certaine culture ? Revue des sciences philosophiques et théologiques, 86, 69-84. doi:10.3917/rspt.861.0069
- Harrison R.G., 1907. Observations on the living developing nerve fiber. The Anatomical Record. 1, 116-128. doi:10.1002/ar.1090010503
- Hooke R., 1965. Micrographia or, some physiological descriptions of minute bodies made by magnifying glasses with observations and inquiries thereupon. London: Printed by Jo. Martyn and Ja. Alleftry; 323 p. doi:10.5962/bhl.title.904
- Hull C.W., 2018. Apparatus for production of three‐dimensional objects by stereolitography. US 4575330. https://pubchem.ncbi.nlm.nih.gov/patent/US-6027324-A
- Kant E., 1986. Œuvres philosophiques. Éditions Gallimard, Paris, France, Collection Bibliothèque de la Pléiade, 317, 1632p) Tome III. https://www.la-pleiade.fr/Catalogue/GALLIMARD/Bibliotheque-de-la-Pleiade/OEuvres-philosophiques2
- Kar S.K., Wells J.M., Ellen E.D., te Pas M.F.W.,Madsen O., Groenen M.A.M., Woelders H., 2021. Organoids: a promising new in vitro platform in livestock and veterinary research. Vet Res 52, 43. doi:10.1186/s13567-021-00904-2
- Kim W., Lee H., Lee J, Atala A., Yoo J.J., Lee S.J., Kim G.H., 2020. Efficient myotube formation in 3D bioprinted tissue construct by biochemical and topographical cues. Biomaterials. 2020 Feb; 230:119632. doi:10.1016/j.biomaterials.2019.119632
- Lavazza A., Massimini M., 2018. Cerebral organoids: ethical issues and consciousness assessment. J. Med. Ethics., 44, 606-610. https://doi/10.1136/medethics-2017-104555
- Le Neindre P., Guatteo R., Guemene D., Guichet J.L., Latouche K., 2009. Douleurs animales. Les identifier, les comprendre, les limiter chez les animaux d’élevage. 338p. https://hal.archives-ouvertes.fr/hal-02990906https://www.inrae.fr/sites/default/files/pdf/eca1cfd56e7ebab5c9f7089673179aa4.pdf
- Le Neindre P., Bernard E., Boissy A, Boivin X., Calandreau L., Delon N., Deputte B., Desmoulin-Canselier S., Dunier M., Faivre N., Giurfa M., Guichet J.L., Lansade L., Larrère R., Mormède P., Prunet P., Schaal B., Servière J., Terlouw C., 2017. Animal consciousness. EFSA supporting publication.14:EN-1196. 165pp. doi:10.2903/sp.efsa.2017.EN-1196
- Lovell-Badge R., Anthony E., Barker R.A., Bubela T., Brivanlou A.H., Carpenter M., Charo R.A., Clark A., Clayton E., Cong Y., Daley G.Q., Fu J., Fujita M., Greenfield A., Goldman S.A., Hill L., Hyun I., Isasi R., Kahn J., Kato K., Kim J.S., Kimmelman J., Knoblich J.A., Mathews D., Montserrat N., Mosher J., Munsie M., Nakauchi H., Naldini L., Naughton G., Niakan K., Ogbogu U., Pedersen R., Rivron N., Rooke H., Rossant J., Round J., Saitou M., Sipp D., Steffann J., Sugarman J., Surani A., Takahashi J., Tang F., Turner L., Zettler P.J., Zhai X., 2021. ISSCR Guidelines for Stem Cell Research and Clinical Translation: The 2021 update. Stem Cell Reports.16, 1398-1408. doi:10.1016/j.stemcr.2021.05.012
- Lucey B.P., Nelson‐Rees W.A., Hutchins G.M., 2009. Henrietta Lacks, HeLa cells, and cell culture contamination. Archives Pathol. Laborat. Medi., 133, 1463-1467. doi:10.1043/1543‐2165‐133.9.1463
- Mandrycky C., Wang Z., Kim K., Kim D.H., 2016. 3D bioprinting for engineering complex tissues. Biotechnol Adv. 34, 422-434. doi:10.1016/j.biotechadv.2015.12.011
- Marano F., Hubert P., Geoffroy L., Juin H., 2020. Quelles alternatives en expérimentation animale ? Pratiques et éthique. Collection Savoir-faire. Éditions Quæ, Versailles, France, 186p. ISBN 978-2-7592-3187-4, référence 02740
- Marquis C.P., 2012. Mammalian cell culture. https://www.eolss.net/sample-chapters/C17/E6-58-01-04.pdf
- McGinley L.M., Kashlan O.N., Bruno E.S., Chen K.S, Hayes J.M., Kashlan S.R., Raykin J., Johe K., Murphy G.G., Feldman E.L., 2018. Human neural stem cell transplantation improves cognition in a murine model of Alzheimer's disease. Sci Rep., 8, 14776. doi:10.1038/s41598-018-33017-6
- Meechan P.J., Wilson C., 2006. Use of ultraviolet lights in biological safety cabinets: a contrarian view. Appl. Biosafety. 11, 222-227. doi:10.1177/153567600601100412
- Mironov V., Boland T., Trusk T., Forgacs Markwald R.R., 2002. Organ printing: computer‐aided jet‐based 3D tissue engineering. Trends in Biotechnology. 21, 157-161. doi:10.1016/S0167-7799(03)00033-7
- Miziara I.D., Magalhães A.T.M., Santos M.A., Gomes E.F., Oliveira R.A., 2012. Research ethics in animal models. Braz. J. Otorhinolaryngol., 78,128-131. doi:10.1590/S1808-86942012000200020
- De Montaigne M., 1962. OEuvres complètes. Éditions Gallimard, collection, Bibliothèque de la pléiade Paris, France. https://www.gallimardmontreal.com/catalogue/livre/oeuvres-completes-montaigne-michel-de-9782070103638
- Munsie M., Hyun I., Sugarman J., 2017. Ethical issues in human organoid and gastruloid research. Dev.,144, 942-945. doi:10.1242/dev.140111
- Murphy S.V., Atala A., 2014. 3D bioprinting of tissue and organs. Nature Biotechnol., 32, 773-785. doi:10.1038/nbt.2958
- Nashimoto Y., Hayashi T., Kunita I., Nakamasu A., Torisawa Y., Nakayama M., 2017. Integrating perfusable vascular networks with a three-dimensional tissue in a microfluidic device. Integr. Biol., 9, 506-518. doi:10.1039/c7ib00024c
- Nicholas J.S., 1961. Ross Granville Harrison1870-1959. Biograph. Memoirs National Academy. Sci., 35, 132-162.
- O'Reilly R.J., 2003. Robert Alan Good, MD, PhD. Biol Blood Marrow Transplant. 9, 608-609. doi:10.1016/j.bbmt.2003.08.010
- Orr B., Sutton K., Christian S. Nash T., Niemann H., Hansen L.L., McGrew M. J., Jensen S.R., Vervelde L., 2021. Novel chicken two-dimensional intestinal model comprising all key epithelial cell types and a mesenchymal sub-layer. Vet. Res., 52, 142. doi:10.1186/s13567-021-01010-z
- Pain B., 2021. Organoids in domestic animals: with which stem cells? Vet. Res., 52, 38. doi:10.1186/s13567-021-00911-3
- Pain B., Baquerre C., Coulpier M., 2021. Cerebral organoids and their potential for studies of brain diseases in domestic animals. Vet. Res., 52, 65. doi:10.1186/s13567-021-00931-z
- Petroianu A., 1996. Aspectos éticos na pesquisa em animais. Acta Cir. Bras., 11, 157-64. https://pesquisa.bvsalud.org/portal/resource/pt/lil-182632
- Plutarque, 2002. Manger la chair ? Traité sur les animaux. Éditions Rivages poche/Petite Bibliothèque Paris, France, 112p. https://www.payot-rivages.fr/rivages/livre/manger-la-chair-9782743643164
- Porphyre, 1979. De l’abstinence. Texte traduit par Bouffartigue J., Patillon M., (Ed.) Belles Lettres. Éditions Belles Lettres, Paris, France, Livre III. https://rspa.ups2259.vjf.cnrs.fr/01/reference-bibliographique/par-id/f7cd76bbf95a5f77117063c83eea47dc8e841bec
- Rimann M., Graf‐Hausner U., 2012. Synthetic 3D multicellular systems for drug development. Current Opinion Biotechnol., 23, 803-809. doi:10.1016/j.copbio.2012.01.011
- Rodriguez‐Hernandez C.O., Torres‐Garcia S.E., Olvera‐Sandoval C., Ramirez‐Castillo F.Y., Muro A.L., Avelar‐Gonzalez F.J., Guerrero‐Barrera A.L., 2014. Cell culture: history, development and prospects. Int. J. Current Res. Academic Review. 12, 188-200.
- Rossi G., Manfrin A., Lutolf M.P., 2018. Progress and potential in organoid research. Nat. Rev. Genet., 19, 671-687. doi:10.1038/s41576-018-0051-9
- Sanchez A., Plouzeau M., Rault P., Picard M., 2000. Croissance musculaire et fonction cardio-respiratoire chez le poulet de chair. (Eds). INRA Prod. Anim., 13, 37-45. doi:10.20870/productions-animales
- Sander K., 1991. Wilhelm Roux and the rest: Developmental theories 1885-1895. Roux’s Arch. Dev. Biol., 200, 297-299. doi:10.1007/BF00665523
- Sato T., Vries R.G. Snippert H.J., van de Wetering M., Barker N., Stange D.E., van Es. J.H., Abo A., Kujala P., Peters P.J., Clevers H., 2009. Single Lgr5 stem cells build crypt–villus structures in vitro without a mesenchymal niche. Nature, 459, 262-265. doi:10.1038/nature07935
- Singer P., 2012. La libération animale. Payot, Éditions, Collection Petite Bibliothèque Payot, 480p. https://www.payot-rivages.fr/payot/livre/la-lib%C3%A9ration-animale-9782228908146
- Smyth D.H., 1978. Alternatives to Animal Experiments– A scolar paperback. Scolar Press [for] the Research Defence Society, ISBN: 0859673952, 9780859673952. 218p
- Souci L., Denesvre C., 2021. 3D skin models in domestic animals. Vet. Res., 52, 21. doi:10.1186/s13567-020-00888-5
- Soupault R., 1952. Vie d'Alexis Carrel 1873-1944, Ouvrage écrit par un chirurgien ayant fait partie du Groupe collaboration, Plon (Eds).
- Souza A.G., Ferreira I.C.C., Marangoni K., Bastos V.A.F., Goulart V.A., 2016. Advances in cell culture: more than a century after cultivating cells. J. Biotechnol. Biomaterials. 6, 1-4. doi:10.4172/2155‐952X.1000221
- Storb R., 2012. Edward Donnall Thomas (1920-2012). Nature. 491, 334. doi:10.1038/491334a
- Sykova E., Forostyak S., 2013. Stem cells in regenerative medicine. Laser Therapy. 2, 87-92. doi:10.5978/islsm.13-RE-01
- Takahashi K., Yamanaka S., 2006. Induction of pluripotent stem cells from mouse embryonic and adult fibroblast cultures by defined factors. Cell. 126, 663-676. doi:10.1016/j.cell.2006.07.024
- Tango C.N., Park J.H., Oh D.H., 2015.An experimental validated in silico model to assess Staphylococcus aureus growth kinetics on different pork products. J. Appl. Microbiol., 120, 684-696. doi:10.1111/jam.13028
- Tannenbaum J., Bennett B.T., 2015. Russell and Burch's 3Rs then and now: the need for clarity in definition and purpose. J. Am. Assoc. Lab. Anim. Sci., 54, 120-132.
- Trounson A., DeWitt N.D., 2013. Pluripotent stem cells from cloned human embryos: success at long last. Cell Stem Cell, 12, 636-638. doi:10.1016/j.stem.2013.05.022
- Vermeire B., Gonzalez L.M., Jansens R.J.J., Cox E., Devriendt B., 2021. Porcine small intestinal organoids as a model to explore ETEC–host interactions in the gut. Ve.t Res., 52, 94. doi:10.1186/s13567-021-00961-7
- Vlaski-Lafarge M., Chevaleyre J., Cohen J., Lafarge X., Ivanovic Z., 2019. Le plasma de sang placentaire, une alternative au sérum de veau fœtal Transfusion Clinique et Biologique 26, S104. doi:10.1016/j.tracli.2019.06.228
- Wu Y.C., Wang Y.C., Wang W.T., Wang H.D., Lin H.H., Su L.J., Kuo Y.R., Lai C.S., Ho M.L., Yu J., 2019. Fluorescent Nanodiamonds Enable Long-Term Detection of Human Adipose-Derived Stem/Stromal Cells in an In Vivo Chondrogenesis Model Using Decellularized Extracellular Matrices and Fibrin Glue Polymer. Polymers, 11. doi:10.3390/polym11091391
- Xiang Y., Miller K., Guan J., Kiratitanaporn W., Tang M., Chen S., 2022. 3D bioprinting of complex tissues in vitro: state-of-the-art and future perspectives. Arch. Toxicol., 96, 691-710. doi:10.1007/s00204-021-03212-y
- Yeager A., 2018. As Brain Organoids Mature, Ethical Questions Arise. The Scientist, 1er août 2018. https://www.the-scientist.com/features/brain-organoids-mature--raise-ethical-questions-64533
Abstract
In France, the use of animals for scientific purposes has been regulated for more than 25 years. Its evolution takes place mainly within a European framework. Directive 86/609 thus aimed to harmonize practices between the Member States. In France as in Europe, regulatory texts on animal protection and the use of animals in experimentation are increasingly demanding. Directive 2010/63/EU of the European Parliament and of the Council of 22 September 2010 relating to the protection of animals used for scientific purposes has greatly reinforced the requirements vis-à-vis animal use. The new directive 2010/63/EU of the European Parliament and of the Council of the 22nd of September 2010 focuses more particularly on measures for reducing animals used for scientific purposes, this use "remaining necessary to protect human health, animal health and the environment". The regulatory corpus associated with the regulations on ethics and animal welfare makes the reduction in the number of animals in experimentation a major challenge for the scientific society. Modern cell culture methods, in silico and modelling methods currently make it possible to reduce the number of animals in animal experiments and to be complementary to these experiments. These methods are booming and there is still a lot of progress to be made in order to be able to answer more scientific questions by alternative methods.
Attachments
No supporting information for this article##plugins.generic.statArticle.title##
Views: 3674
Downloads
PDF: 44
XML: 11
Most read articles by the same author(s)
- Frédéric DESSAUGE, Cindy SCHLEDER , Marie-Héléne PERRUCHOT, Karl ROUGER, 3D in vitro models of skeletal muscle: innovative approaches for animal science , INRAE Productions Animales: Vol. 36 No. 2 (2023): Cellules souches et Organoïdes : réalités et perspectives